Mechanics of fiber-reinforced concrete failure:
Laboratory study of the effect of gender, geometry and composition of different fibers
Khalid Sanginabadi1, Ruholah Rostami2, Bardah Habibi3, Daoud Mostufinejad4*, Mohammad Zarebini
1- PhD student in civil engineering, Faculty of Civil Engineering, University of Kurdistan, Sanandaj, Iran
2- Doctoral student of textile engineering, faculty of textile engineering, Isfahan University of Technology, Isfahan, Iran
3- Assistant Professor, Faculty of Mechanical Engineering, University of Kurdistan, Sanandaj, Iran
4-Professor, Faculty of Civil Engineering, Isfahan University of Technology, Isfahan, Iran
5- Associate Professor, Faculty of Textile Engineering, Isfahan University of Technology, Isfahan, Iran
Abstract
In recent years, the use of polymer fibers has seen significant growth in concrete reinforcement. The main role of fibers comes into play in the post-cracking behavior of concrete. A detailed investigation of the cracking behavior requires the application of fracture mechanics concepts in the process of fracture and crack propagation in concrete beams. For this purpose, 14 concrete specimens with dimensions of 400×100×100 millimeters, containing a 35-millimeter deep notch at their center of tension face, were subjected to four-point bending tests. In these specimens, different types of fibers, geometry, and percentage of fiber combinations were considered as variables.
The results indicate that macro polypropylene fibers, despite increasing flexural strength, do not have a significant influence on the ductility of concrete. Micro polyester and polypropylene fibers show better performance during concrete cracking, and for specimens containing these fibers, no sudden drop in load-carrying capacity occurs at the onset of cracking. Furthermore, the results of composite specimens containing macro polypropylene and micro polyester fibers showed that although the flexural strength of the cracking increases with the increase in the percentage of macro fibers, the ductility indices decrease proportionally.
Considering all the characteristics, the specimen with a ratio of 3 to 2 of macro polypropylene fibers to micro polyester fibers exhibits the best performance.
Keywords: Fracture mechanics, Fiber-reinforced concrete, Polypropylene, Polyester, Ductility.
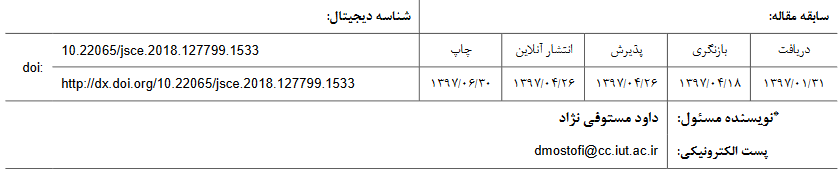
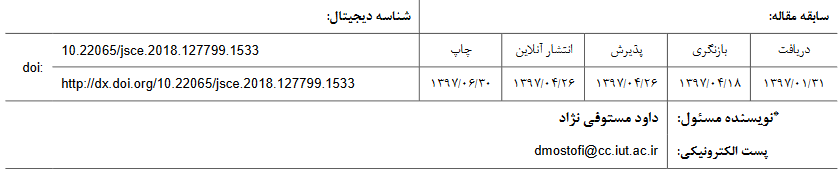
Fracture mechanics of fiber reinforced concrete: Experimental study of
composition, geometry and hybridization of fibers
Khaled Sanginabadi1, Rohallah Rostami2, Nabard Habibi3, Davood Mostofinejad4*, Mohammad
Zarrebini5
1- PhD candidate in Structural Engineering, Department of Civil Engineering, University of Kurdistan, Sanandaj, Iran
2- PhD candidate in Textile Engineering, Department of Textile Engineering, Isfahan University of Technology, Isfahan, Iran
3- Assistant Professor, Department of Mechanical Engineering, University of Kurdistan, Sanandaj, Iran
4- Professor, Department of Civil Engineering, Isfahan University of Technology, Isfahan, Iran
5- Associate Professor, Department of Textile Engineering, Isfahan University of Technology, Isfahan, Iran
ARTICLE INFOABSTRACT
Received: 20/04/2018
Revised: 09/07/2018
Accepted: 17/08/2018
Using of polymeric fibers for reinforced concrete structures has
significantly developed in recent years. Polymeric fibers start their
contribution in the behavior of concrete members after cracking. In order
to a careful investigation into post-cracking behavior need to apply fracture
mechanic concept (growth of cracks) in reinforced concrete members. For
this purpose, in this research 14 concrete prisms (100×100×400 mm in
dimensions) with 35 mm notch depth (at the center of tensile side) for four-
point flexural strength test were fabricated. Fiber composition, geometry
and hybridization percent were varied in these samples. Derived outputs
illustrated that macro polypropylene (PP) fiber has no significant effect on
concrete ductility, whereas it leads to increase the flexural strength. But
micro polyester (PET) and PP fibers have more effective performance
during forming cracks in concrete members. PET and PP fibers have a
more suitable function during concrete cracking and the samples
containing these fibers have no significant drop in their bearing while the
cracking is started. In addition, samples reinforced with PP and PET fibers
indicated that by increase in macro fibers, the flexural strength were
increased where as ductility indices decreased. In general, samples
reinforced with %60 of PP macro fibers and %40 PET micro fibers have
the best performance
.
Keywords:
Fracture Mechanics
FRC
Polypropylene
Polyester
Ductility
All rights reserved to Iranian Society of Structural Engineering.
doi: 10.22065/jsce. 2018.127799.1533
*Corresponding author: Davood Mostofinejad.
Email address: dmostofi@cc.iut.ac.ir
Introduction
The advantages of reinforced concrete structures over other types of structures have made concrete one of the most widely used construction materials today. Besides its suitable compressive strength, concrete exhibits ductility, tensile strength, and flexural strength. In recent years, significant improvements have been made by adding polymer fibers to concrete and producing fiber-reinforced concrete. The main advantage of fibers lies in their performance during concrete cracking. In fiber-reinforced concrete, fibers are randomly distributed in different directions and act as bridges across crack faces, preventing crack initiation and propagation [1].
Concrete cracks are classified into micro and macro cracks based on their scale. The propagation and cohesion of micro cracks give rise to macro cracks. Therefore, it is beneficial to use fibers in two different scales in concrete. Micro fibers, with a length of less than 30 millimeters, are responsible for crack control but do not significantly contribute to improving the tensile and flexural properties of concrete due to their lower mechanical properties compared to macro fibers. On the other hand, macro fibers, with appropriate mechanical properties, not only control macro cracks but also enhance the mechanical properties of concrete [2].
The performance of fibers during concrete cracking depends on various factors, including the type of fibers, fiber length, fiber cross-sectional area, fiber orientation, and fiber-matrix bond. Many research studies have evaluated the effect of these factors using different methods and tests. Considering the mechanisms of concrete cracking, fracture mechanics-based approaches can be useful. Therefore, numerous laboratory and analytical studies have been conducted based on fracture mechanics concepts [2].
In 2007, Huan et al. investigated the fracture behavior of fiber-reinforced concrete members using artificial fibers. They first optimized the fiber geometry and then experimentally and theoretically studied the crack resistance and post-cracking behavior of fiber-reinforced concrete. The results indicated that the load-CMOD and displacement-CMOD curves obtained from their model were very close to the experimental results [3].
In 2010, Bencardino et al. conducted an experimental study on the fracture characteristics of fiber-reinforced concrete. They used polypropylene fibers with a length of 19 millimeters and wollastonite fibers with a length of 50 millimeters. The results showed that the fracture energy was related to the amount of fibers used, with a greater increase observed for wollastonite fiber-reinforced concrete [4].
In 2012, Caggiano et al. investigated the fracture behavior of concrete beams reinforced with a combination of micro and macro wollastonite fibers. While the crack resistance and post-cracking behavior were strongly related to the volume of fibers, the combination of micro and macro fibers had no significant influence on crack resistance and ductility after cracking [5].
Alberti et al. conducted an extensive study in 2016 on the fracture mechanics of concrete reinforced with polyvinyl alcohol fibers. They investigated the properties of concrete, casting direction, fiber length, and specimen size. The results showed that using longer fibers compensated for fiber pull-out due to better orientation and distribution, but the fiber length had no significant effect on residual strength and only slightly reduced CMOD [6].
Despite extensive research, due to the uncertainty of results and the numerous parameters affecting concrete cracking behavior, further research seems necessary. Therefore, this study aims to investigate the fracture mechanics of concrete beams reinforced with fibers through laboratory experiments. The parameters under consideration include fiber type, fiber geometry, and various combinations of fibers with different types and geometries. Load-displacement, load-CMOD, and load-Crack Tip Opening Displacement (CTOD) curves were extracted for the tested specimens. These curves were then used to calculate and analyze relevant fracture behavior parameters.
To measure displacement fields, Particle Image Velocimetry (PIV), a digital image processing-based technique, was used. PIV has been employed in fluid mechanics and soil mechanics studies for displacement measurements. Hussein et al. used this technique for the first time in structural research in 2012 [7].
To investigate the post-cracking behavior of fiber-reinforced concrete, standardized specimens, test arrangements, and specific standard conditions were defined. These standard conditions are proposed by various national and international regulations. Among the most important of these regulations are UNI 11039-2 and EN 14651. The mentioned standards introduce three-point or four-point bending tests for beams with a crack on the tensile face. Accordingly, in this study, the four-point bending test with a crack on the tensile face was used [8,9].
Laboratory Procedures
2.1. Specimen Specifications and Concrete Mixing Design
For comparing the effect of macro polypropylene and micro polyester fibers on the behavior of fiber-reinforced concrete, seven different concrete mixes were prepared. Each mix included two cylindrical and two prismatic specimens. The prismatic specimens were of dimensions 400×100×100 millimeters for performing the four-point bending test, and the cylindrical specimens had dimensions of 300×150 millimeters for determining the tensile strength of each mix. Additionally, as shown in Figure 1(a), a 35-millimeter deep crack was created at the center of the tensile face of the prismatic specimens.
The concrete mixing was performed according to the ACI-211 standard. The concrete mixing proportions included 379 kilograms per cubic meter of special type 1 cement, 757 kilograms per cubic meter of coarse aggregate, 917 kilograms per cubic meter of fine aggregate, and 220 kilograms per cubic meter of water. The largest dimension of the coarse aggregate was 5.9 millimeters, and the largest dimension of the fine aggregate was 4.75 millimeters. After mixing, the concrete was cast in metal molds for prismatic and cylindrical specimens. The molds were opened after 24 hours, and the specimens were cured under standard laboratory conditions for 28 days. Once the curing was completed, a suitable color pattern (white, blue, red, green, and black) was applied to the surface of the specimens at one-third of their length under pure bending conditions (middle span) using the PIV method to achieve accurate displacement fields.
2.2. Fiber Specifications
Polypropylene and polyester fibers were used with a constant dosage of 6 kilograms per cubic meter. The micro fibers were prepared with lengths of 18 millimeters and diameters of 12 micrometers. The macro fibers were also of polypropylene and had lengths of 33 millimeters. The properties of the used fibers were measured according to the ASTM D 3822 standard and are presented in Table 1.


2-3. Sample Specifications and Classification
The samples were prepared in two main series. The first series consisted of fibers of a single type and length, while the second series included a combination of fibers of two different types and lengths. The nomenclature for the first series is in the form of X-Y-n. If the fibers are micro fibers, X is represented as “micro,” and if they are macro fibers, X is represented as “macro.” Y indicates the type of fiber, where “PET” represents polyester fibers, and “PP” represents polypropylene fibers. n represents the test repetition number (1 or 2).
The second series of samples was prepared by blending two types of fibers. The nomenclature for these samples is in the form of “blend-x-y-n.” X indicates the percentage of macro polypropylene fibers in the total fiber content, and Y indicates the percentage of micro polyester fibers in the total fiber content. n represents the test repetition number (1 or 2). For example, the sample “blend-80-20-1” indicates that it is a composite sample with 80% of its fibers being macro polypropylene and 20% being micro polyester. It is also the first repetition of the test.
2-4. Testing Equipment
The four-point bending test was conducted at Isfahan University of Technology using an ELE brand TavFens machine with a capacity of 50 kilo newtons (Figure 1). In all the samples, the bending loading was applied using controlled displacement at a speed of 0.3 millimeters per minute.
The tensile splitting strength of concrete samples was measured using the Brazilian test. For this purpose, the samples were placed inside a special frame and loaded using an ELE brand hydraulic jack with a capacity of 2,000 kilo newtons.
To capture sequential images of the specimen surfaces during the test and for PIV technique, a Nikon 5200 camera with a resolution of 24 megapixels equipped with a Nikkor 18-135 mm lens was used.
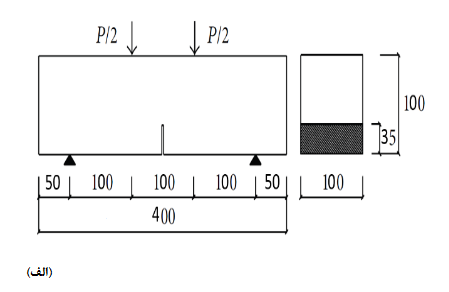
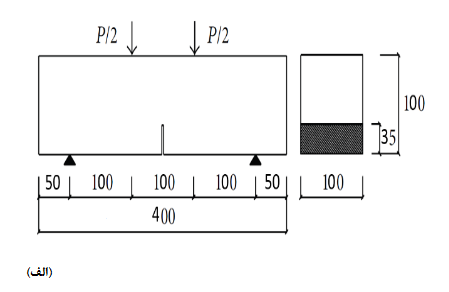
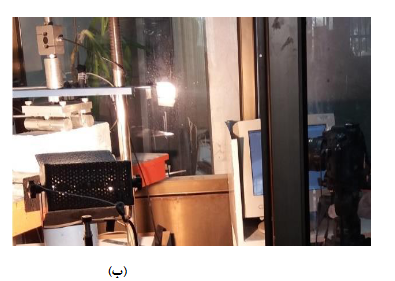
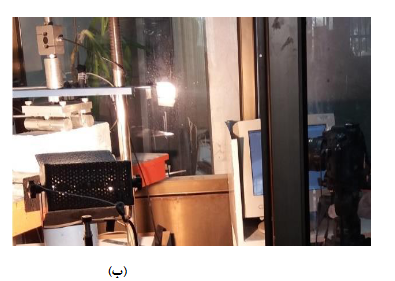
Figure 1: (a) Dimensions and loading procedure; (b) Four-point bending test apparatus and imaging equipment components.
3- Results and Discussion
The results of the bending and tensile tests for all samples are presented in Table 2. The tensile strength was measured for each sample on the day of the bending test. Figure 3 shows the comparison of ultimate load and tensile strength for better understanding of the results. It is evident that macro fibers have a significant impact on the tensile strength and ultimate load-carrying capacity of the beams. For example, the macro-PP sample, which contains all macro fibers, has approximately 89% and 99% higher tensile strength and ultimate load-carrying capacity compared to the micro-PET sample. Moreover, as the percentage of micro polyester fibers increases relative to macro polypropylene fibers, the tensile strength and ultimate load-carrying capacity decrease. For instance, the blend-80-20 sample, with 80% of its total fibers being macro polypropylene and 20% being micro polyester, has approximately 31% and 22% higher tensile strength and ultimate load-carrying capacity than the blend-20-80 sample (with 80% micro polyester fibers and 20% macro polypropylene fibers).
Furthermore, the comparison between the micro-PP and micro-PET samples indicates that polypropylene fibers exhibit better performance in the tensile and flexural behavior of concrete. Figure 4 illustrates the cracking of a specimen at the final moment.
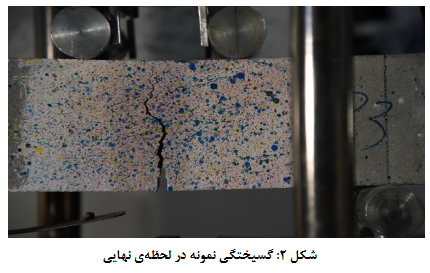
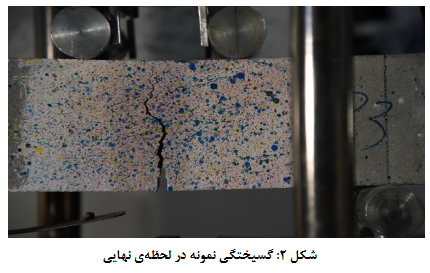
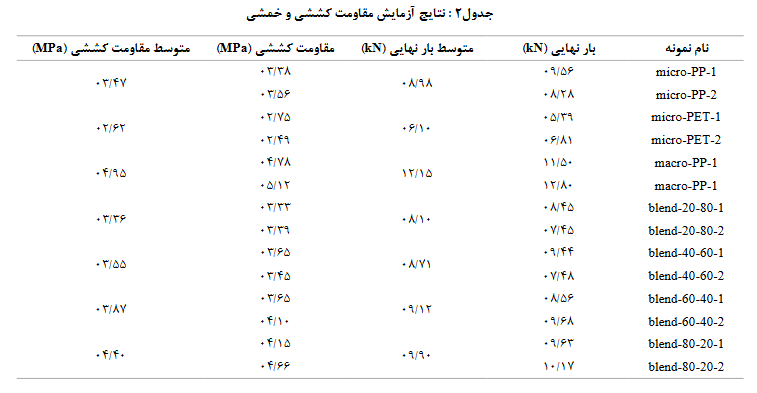
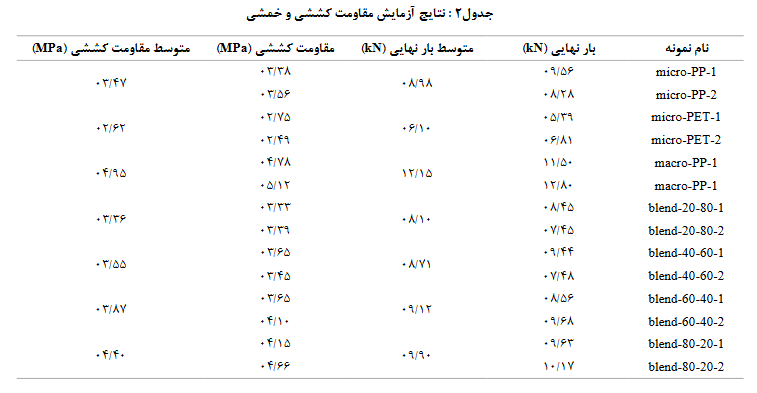
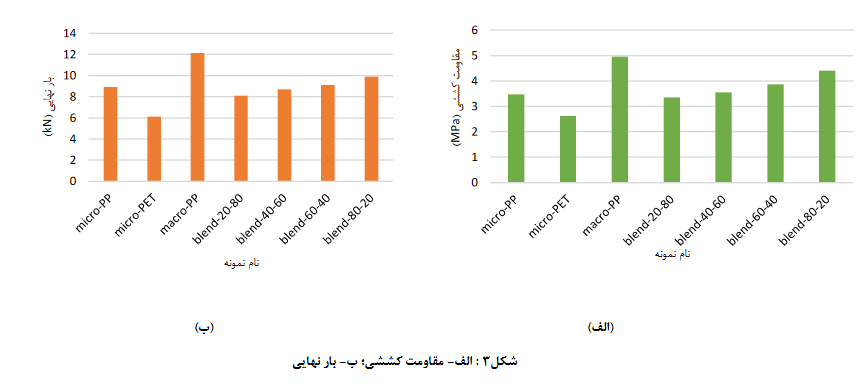
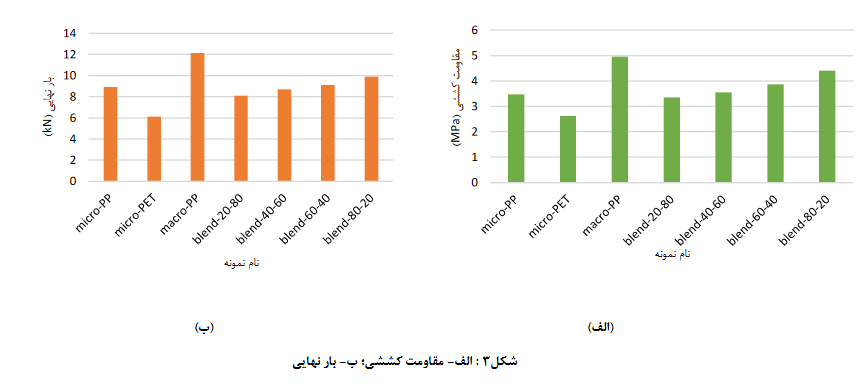
3-1 Behavior of Fiber-Reinforced Concrete in Fracture
Cracking in the middle of the beam and opening of the crack tip and mouth are parameters influencing the behavior of fiber-reinforced concrete beams. By plotting load-displacement curves, load-CMOD (Crack Mouth Opening Displacement), and load-CTOD (Crack Tip Opening Displacement) curves, these effects are investigated. For this purpose, image processing using PIV (Particle Image Velocimetry) is employed to accurately assess all displacements and deformations. To evaluate displacements and deformations, the middle one-third of the beam (pure bending region) is speckled. To eliminate possible movement of the concrete beam and local deformations at supports, one 128×128 pixel patch is placed at the middle one-third of the beam, and two similar patches are placed on each side of the middle part of the beam. This way, all displacements in the middle one-third of the beam can be obtained. Moreover, by recording the load at each moment using a load cell, the mentioned curves can be plotted. These curves are extracted for all samples, and for a more appropriate analysis of the curves, the average curve of the first and second repetitions is plotted for each sample.
3-1-1 Effect of Fiber Material and Geometry
Based on Figure 4, samples containing micro polyester, micro polypropylene, and macro polypropylene fibers exhibit different behavior and failure modes. The load-carrying capacity of macro polypropylene (macro-PP) samples is higher compared to micro polypropylene (micro-PP) and micro polyester (micro-PET) samples. The load-carrying capacity of macro-PP samples decreases after the first crack, while micro-PP and micro-PET samples show a gradual decrease in load after the first crack occurrence. At the final moment, the load-carrying capacity of macro-PP, micro-PP, and micro-PET samples is approximately 2 kN, 0.7 mm, 9.5 mm, and 4.5 mm CMOD (Crack Mouth Opening Displacement), respectively.
Figure 4(b) indicates better performance of the micro-PET sample compared to micro-PP and macro-PP samples. At the final moment, the CMOD value for the micro-PET sample is approximately 5.6 mm, whereas it is 9.6 mm and 8.8 mm for micro-PP and macro-PP samples, respectively. Additionally, Figure 4(c) shows the better performance of the micro-PET sample. The CTOD (Crack Tip Opening Displacement) values for micro-PET, micro-PP, and macro-PP samples are approximately 7.5 mm, 5.6 mm, and 8.7 mm, respectively. Therefore, the CMOD and CTOD values for the micro-PET sample are about 26% and 27% less than those for the macro-PP sample, respectively. These values are approximately 5% and 12% less than those for the micro-PP sample, respectively.
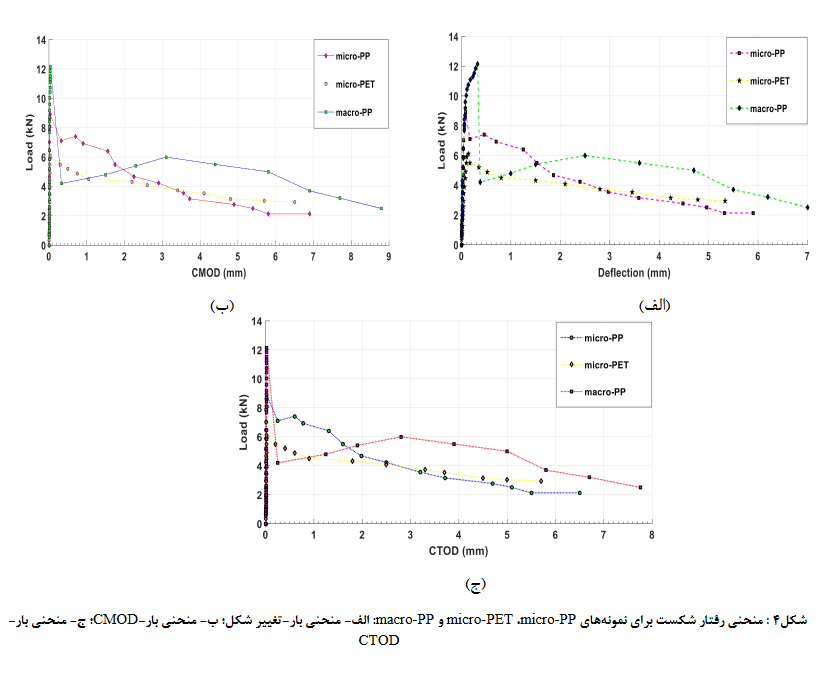
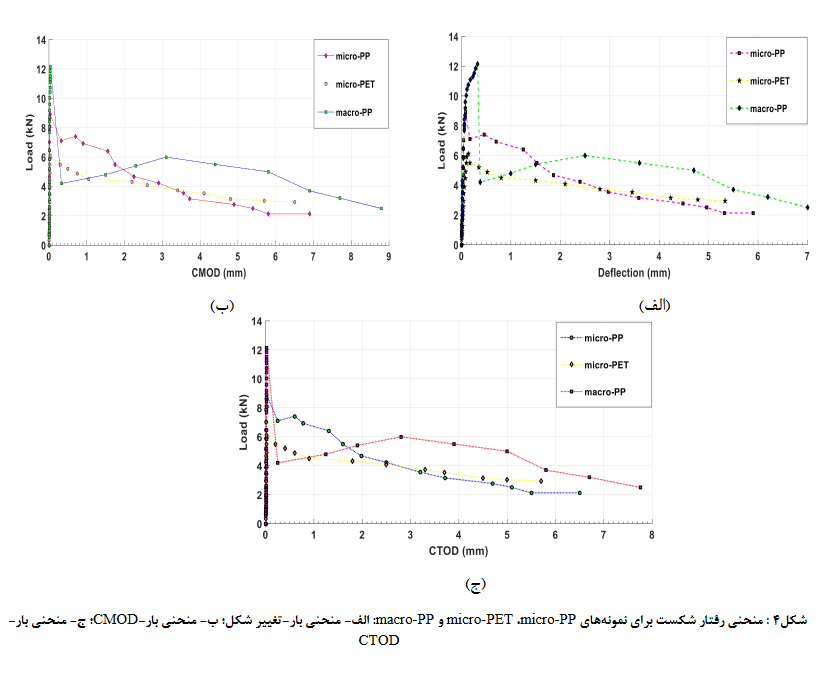
3-1-2 The effect of fiber composition
Figure 5 shows the comparison of the fracture behavior of samples with different amounts of micro polyester and macro polypropylene fibers. Based on this figure, as the amount of macro fibers increases (and micro fibers decrease); Sudden failure and a noticeable drop in load are more apparent. So that the blend-20-80 sample (20% macro fibers and 80% micro fibers) has a 42% less load loss than the blend-80-20 sample (80% macro fibers and 20% micro fibers). A similar trend is observed for the final amount of CTOD. Meanwhile, with the increase in the amount of micro polyester fibers, the final value of CMOD has a slight decreasing trend. The blend-60-40 sample (60% polypropylene macro fibers, 40% micro polyester fibers) compared to the micro-PET sample (100% micro polyester fibers) shows a 59% increase in CTOD. This number for CMOD value is 50%.
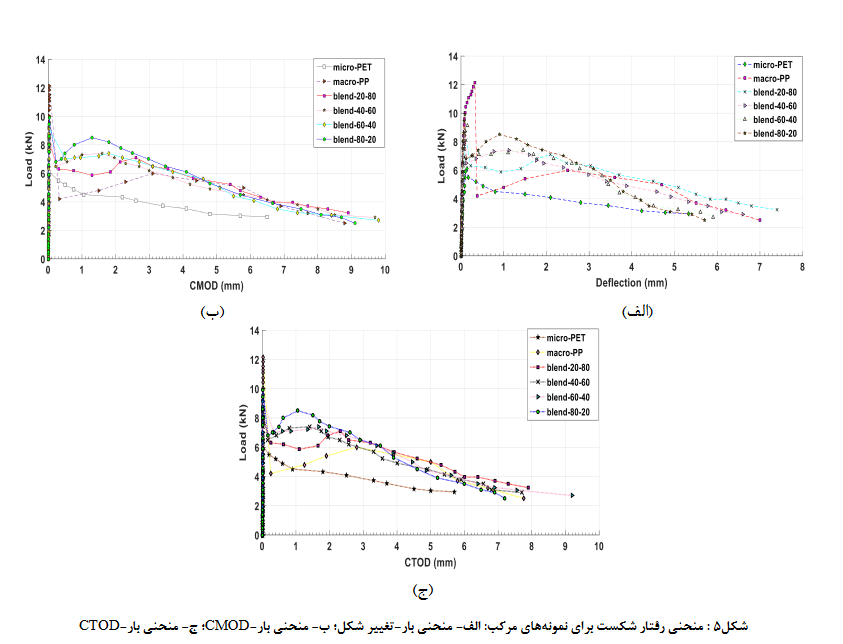
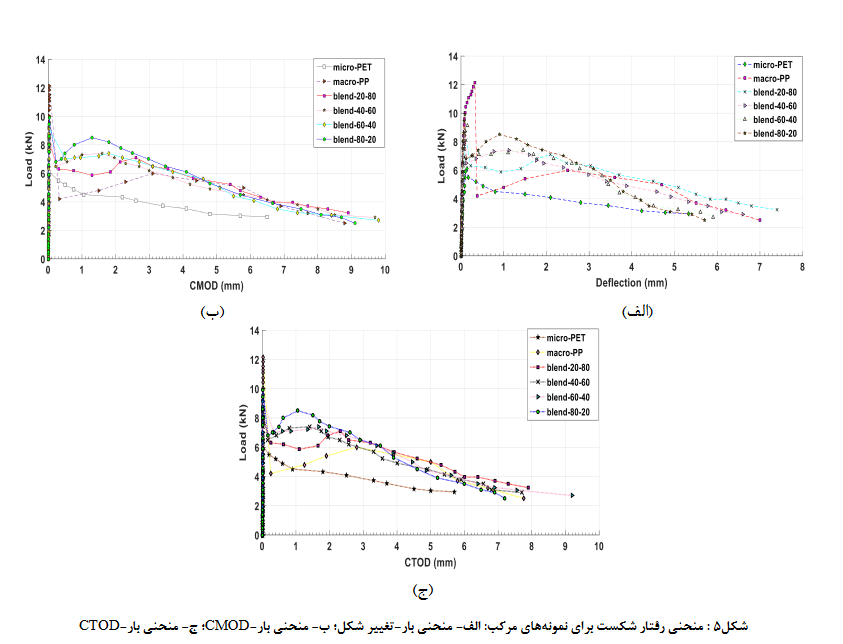
3-2 fiber concrete fracture parameters
3-2-1 based on UNI 11039-2 [8]
UNI 11039-2[8] defines parameters for evaluating the behavior after cracking of concrete beams. These parameters help
Load-deformation and load-CTOD diagrams are calculated. As the first definition, we can refer to the nominal cracking resistance
It is defined according to relation 1.


In relation (1), IfP is the load corresponding to cracking (Newton), b is the width of the sample (100 mm), h is the height of the sample (100 mm), l is the length
The sample is (300 mm) and the gap height is (35 mm). Also, to express the average cracking resistance for CTOD in the range of cracking initiation (0 CTOD) to CTOD equal to 0.6 mm (CTOD 0.6) and CTOD between 0.6 and 3 mm, two parameters are defined in the form of relations (2) and (3), respectively.




In these relationships, all parameters are defined similar to relationship (1). Also, parameters 1U and 2U according to Figure 6 and relations (4) and
(5) are calculated. This regulation provides 0D and 1D malleability indices to express the degree of ductility (relationships (6) and (7) respectively).
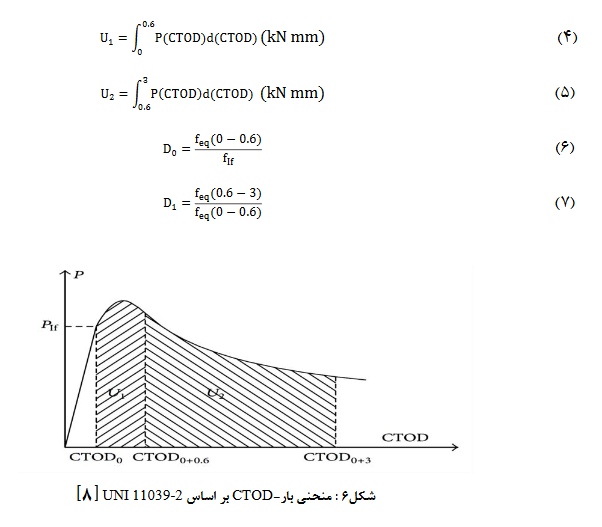
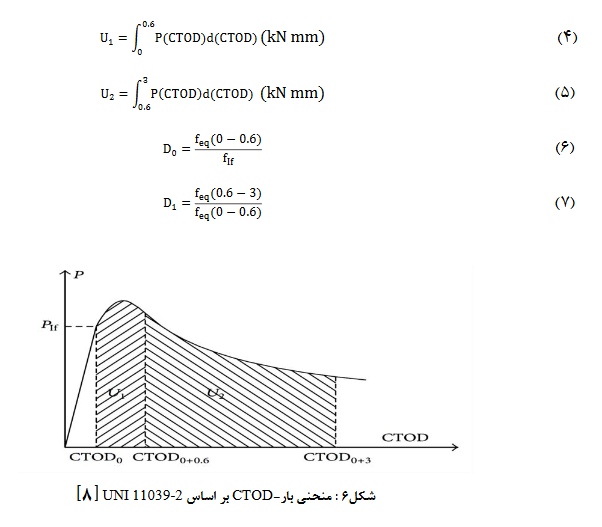
Average parameters related to relationships 1 to 7 were calculated for all samples and presented in Figure 7. According to this figure, although the micro-PP sample has higher cracking resistance; But it does not have significant energy absorption and plasticity factors. Meanwhile, blend samples perform better than non-composite samples in terms of plasticity and energy absorption factors. According to all the discussed parameters and based on Figure 7, the result of the characteristics of the blend-60-40 sample is more suitable than other samples.
3-2-2 based on EN 14651 [9]
EN 14651[9] defines indicators to evaluate the post-cracking behavior of concrete beams under bending test. These parameters are extracted with the help of load-deformation and load-CMOD diagrams. As the first definition, we can refer to the proportionality limit resistance
show (relationship (8).)


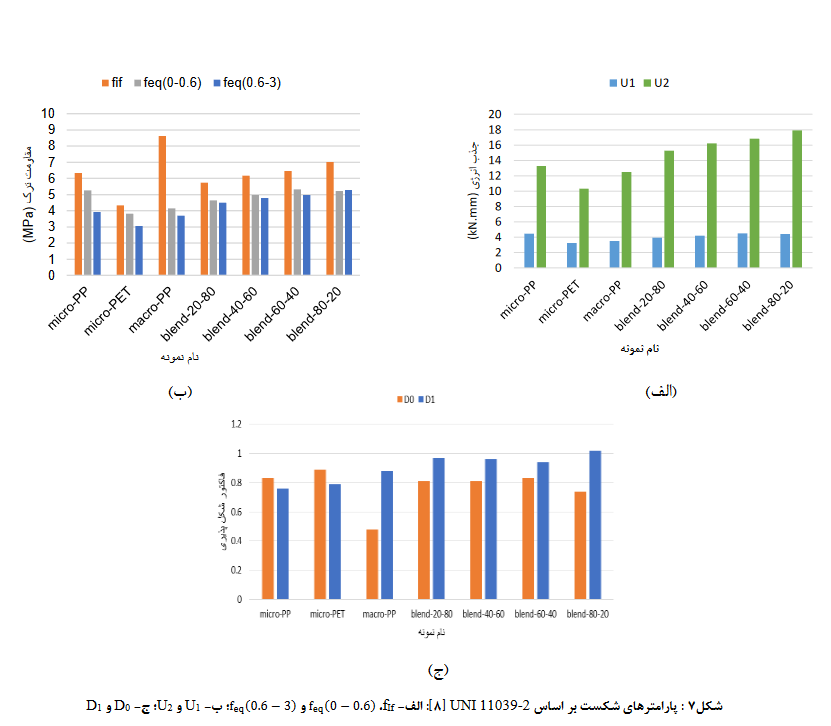
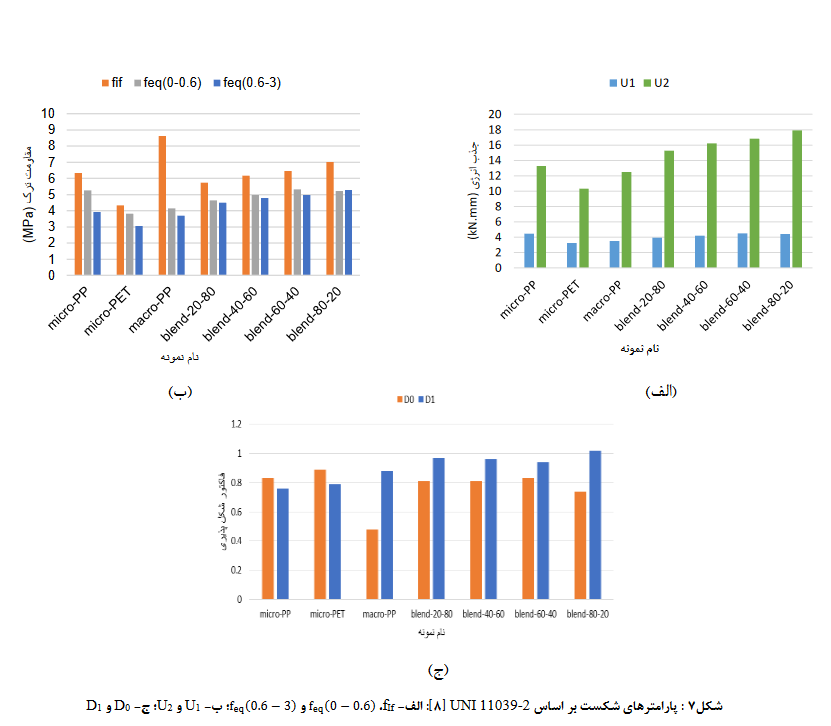
In relation (9) LF, the maximum parallel load with CMOD is equal to 0.05 mm, b sample width (100 mm), l sample length (300 mm
m) and sph is the distance between the tip of the crack and the top of the sample (65 mm). Also, the values of residual bending strength corresponding to CMOD are equal
0.5, 1.5, 2.5, and 3.5 mm are indicated by indices 1R, f, 2R, f, 3R, f, and 4R, f and are calculated through relation (9). Define parameters
Relationship (9) is similar to relationship (8) and determination of R, jF is based on Figure 8. The strength of the fit limit and the mentioned indices for all samples
The calculation and its average values are shown in Figure 9.


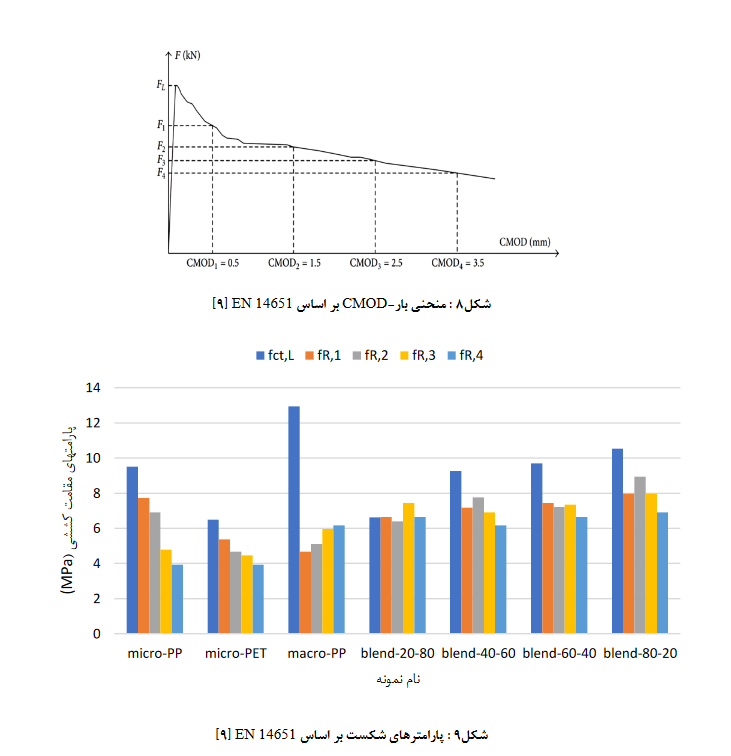
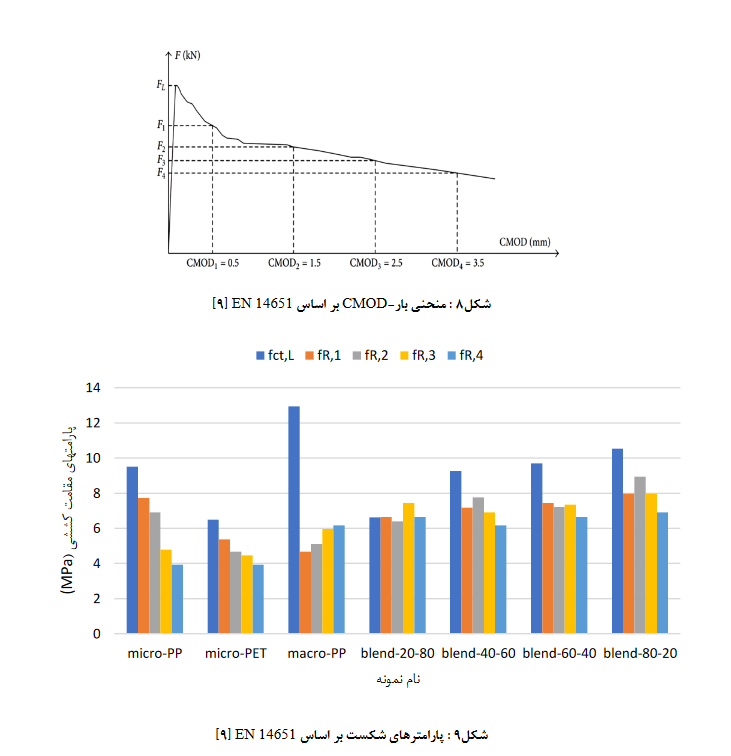
Figure 9 shows that the macro-PP sample has a very suitable limit resistance compared to other samples. So that the resistance of this sample shows a 100% increase compared to micro-PET and blend-20-80 samples. Meanwhile, in residual stress indices, blend-80-20 and blend-60-40 samples have a much better performance than other samples, especially PP-macro and PET-micro samples. So that index 2R, f in 20-80-blend sample is increased by more than 100% compared to PET-micro sample. Figure 9 shows that the plasticity performance of blend samples is more suitable than non-composite samples.
4- Conclusion
In this study, to investigate the fracture behavior of fiber-reinforced beams using fracture mechanics concepts, 14 prismatic specimens were fabricated and tested. The variables considered were the fiber material, fiber geometry, and fiber combination method. The summary of the research results is as follows:
1- Specimens containing macro polypropylene fibers exhibit higher crack resistance and tensile strength compared to specimens containing micro polyester and polypropylene fibers, or a combination of macro and micro fibers.
2- Fiber-reinforced concrete containing micro polypropylene fibers shows better flexural and tensile performance compared to fiber-reinforced concrete containing micro polyester fibers.
3- In the case of concrete reinforced with macro polypropylene fibers, despite higher load-carrying capacity compared to concrete reinforced with micro polypropylene, polyester, or a combination of macro and micro fibers, an abrupt decrease (up to 67%) in load-carrying capacity occurs after the initiation of cracking, leading to sudden failure. In contrast, the other specimens experience a gradual failure mode with no significant sudden drop in load-carrying capacity.
4- Specimens with all micro polyester fibers show better performance in terms of crack mouth opening displacement (CMOD) and crack tip opening displacement (CTOD) compared to specimens with a combination of macro and micro polypropylene fibers. The CMOD value for these specimens is reduced by 26% and 5% compared to specimens containing macro and micro polypropylene fibers, respectively. The corresponding CTOD values are 27% and 12%.
5- In terms of workability factors, specimens containing 60% macro polypropylene fibers and 40% micro polyester fibers, and specimens containing 80% macro polypropylene fibers and 20% micro polyester fibers, show significantly better performance than other specimens. These mentioned specimens are identified as having the optimal fiber combination percentage.
References
[1] di Prisco, M. Plizzari, G. Vandewalle, L. (2009). Fiber reinforced concrete: new design perspectives. Materials and
Structures, 42(9), 1261–81.
[2] Afroughsabet, V. (2016). High-performance fiber-reinforced concrete: a review. materials science, Vol. 51, pp. 6517–
6551.
[3] Byung, H. Ji, C. Young, C. (2007). Fracture behavior of concrete members reinforced with structural synthetic fibers.
Engineering Fracture Mechanics, Vol. 74, pp. 243–257.
[4] Bencardino, F. Rizzuti, L. Spadea, G. Swamy, R. (2010). Experimental evaluation of fiber reinforced concrete fracture
properties. Composites Part B: Engineering, Vol. 41, pp. 17–24.
[5] Caggiano, A. Cremona, M. Faella, C. Lima, C. Martinelli, E. (2012). Fracture behavior of concrete beams reinforced with
mixed long/short steel fibers. Construction and Building Materials, Vol. 37, pp. 832–840.
[6] Alberti, M. Enfedaque, A. Gálvez, J. (2016). Fracture mechanics of polyolefin fibre reinforced concrete: Study of the
influence of the concrete properties, casting procedures, the fiber length and specimen size. Engineering Fracture Mechanics,
Vol. 154, pp. 225–244.
[7] Hosseini, A. Mostofinejad, D. Hajialilue-bonab, M. (2012). Displacement and strain field measurement in steel and RC
beams using particle image velocimetry. Engineering Mechanics, Vol. 4, pp. 1–10.
[8] UNI 11039-2. 2003. Steel Fiber Reinforced Concrete—Part2: Test Method for Determination of First Crack Strength and
Ductility Indexes.
[9] EN 12390-3. 2009. Testing Hardened Concrete—Part3: Compressive Strength of Test Specimens.
[10] ACI Committee 211. ACI 211.1-91. 2002. Standard practice for selecting proportions for normal, Heavyweight, and
Mass Concrete, Farmington Hills, MI, USA.
[11] ASTM D 3822. 2014. Standard Test Method for Tensile Properties of Single Textile Fibers.